Structural Biochemistry/Molecular Geometry
Molecular Geometry
Definitions
[edit | edit source]Molecular geometry is the 3-dimensional shape that a molecule occupies in space. It is determined by the central atom and the surrounding atoms and electron pairs. The shape of most molecules can be predicted using the Valence Shell Electron Pair Repulsion (VSEPR) method. This method states a few rules to help one determine the shape of a substance without using high technology methods such as X-ray crystallography, NMR Spectroscopy, or electron microscopy. Some of the most common shapes that can be taken are linear, trigonal planar, tetrahedral, pyramidal, and angular (or bent).
VSEPR Theory
[edit | edit source]Valence Shell Electron Pair Repulsion (VSEPR) theory Electron pairs around a central atom arrange themselves so that they can be as far apart as possible from each other. The valence shell is the outermost electron-occupied shell of an atom that holds the electrons involved in bonding. In a covalent bond, a pair of electrons is shared between two atoms. In a polyatomic molecule, several atoms are bonded to a central atom using two or more electron pairs. The repulsion between negatively charged electron pairs in bonds or as lone pairs causes them to spread apart as much as possible. The idea of "electron pair repulsion can be demonstrated by tying several inflated balloons together at their necks. Each balloon represents an electron pair. The balloons will try to minimize the crowding and will spread as far apart as possible. According to VSEPR theory, molecular geometry can be predicted by starting with the electron pair geometry about the central atom and adding atoms to some or all of the electron pairs. This model produces good agreement with experimental determinations for simple molecules. With this model in mind, the molecular geometry can be determined in a systematic way. Molecules can then be divided into two groups: Group 1: Molecules with NO lone electron pairs. In this case the molecular geometry is identical to the electron pair geometry. Group 2: Molecules with one or more lone electron pairs. In this case an extra step is needed to to translate from electron pair geometry to the final molecular geometry, since only the positions of bonded atoms are considered in molecular geometry.
The VSEPR theory says that:
1) The electron pairs and atoms around a central atom will give a molecule its shape.
2) Electron pairs around a central atom in a molecule will attempt to be as far away from each other as possible.
3) Lone pairs around the central atom will have an effect on the shape of the entire molecule, just like an atom would.
4) Lone pairs of electrons repel each other more strongly than a lone pair and a shared pair, which in turn repel each other more than two shared pairs (or bonds between the central atom and another atom).
These are just a few of the basic postulates of the VSEPR theory that, along with the lewis dot structure of a molecule, allow us to determine the shape it will have.
Ring Molecular Geometry
[edit | edit source]Many organic compounds contain rings of carbon atoms or other atoms such as oxygen or nitrogen. The simplest ring compound contains 3 carbons as in cyclopropane. The most common ring compounds contain either 5 or 6 carbons. These compounds are also called cyclic.
Bent Molecular Geometry
[edit | edit source]The molecule that is made up of 4 equally spaced sp3 hybrid orbitals forming bond angles of approximately 109.5o. The shape of the orbitals is tetrahedral. Two of the orbitals contain lone pairs of electrons. The two atoms connected to the central atom form a molecule with a bent shape.
Hybridization and Geometry
[edit | edit source]There is a simple relationship between the hybridization of an atom and its geometry, i.e., the way or ways in which other atoms can be disposed about it in space. This module examines the allowed bonding patterns for the representative elements B, C, N, O, and F, as well as their geometries as a function of their hybridization and formal charge.
Finding Hybridization For Hybridization of molecules: 1. Count total number of valence Electrons 2. Divide by 8, if no of e- > 8. Divide by 2, if no of e- ≤ 8.
Polarity and Geometry
[edit | edit source]The geometry of a molecule determines many properties such as polarity, reactivity, state, and much more. The polarity is directly related to the shape because many molecules with polar bonds can be non polar as a whole due to symmetry of the molecule that has equal electron pull outwards or inward. One such example is carbon dioxide. The electronegative oxygens on either side of the carbon pull atoms toward them selves, cause a partial negative charge on each end, while the less electronegative carbon is left with a partial positive charge. The oxygens are both double bonded to the carbon leaving no lone pairs to bend the molecule, which is linear and has 180 degree bond angles. The equal pull of both partial negative charges balance each other out because they are of equal magnitude and exact opposite directions and the whole molecule is non polar. .

On the other hand, a molecule can have polar bonds and a different shape that does not balance out the electronegative pull. For example, the hydrogen dioxide molecule, which also contains three atoms but has a different shape. Water is tetrahedral and bent because it has two extra electron pairs which push the hydrogen atoms into a 109.5 degree angle. As a result, the partial negative charge on oxygen is balanced on each side, but is pointed up and inwards, giving the whole molecule polar properties.

The polarity in turn then affects many properties itself, such as melting point, boiling point, vapor pressure, solubility and the types of bonds that can be formed, just to name a few. Polar molecules will have higher melting and boiling points and lower vapor pressure due to the attraction between the molecules. Polar molecules also tend to dissolve other polar molecules while non polar molecules also dissolve other non polar substances. This is often referred to as "like dissolves like" as a quick way to remember this property.
Reactivity and Geometry
[edit | edit source]Another property determined by molecular geometry is reactivity. The reactivity is affected in many ways. One example is the type of bonds the molecule makes based on polarity. For example, some polar molecule can react in hydrogen bonds while non polar molecules react in london dispersion forces. But there are other ways that the geometry directly affects sections that a molecule partakes in. Large bulky molecules often react using the unhindered side, even if it is not as favorable of a product, because of the easier reaction. Another example is enzymes and the way the "fit" certain substrates based on their own sake and the shape of the substrates. These are just a couple examples of the many ways that molecular shape can help determine reactivity.

Determination of Geometry
[edit | edit source]Although VSEPR and a few similar methods can be used to determine the geometry of a molecule, there are high tech methods that can show us the shape of much larger molecules, usually proteins, without a doubt. These methods include X-ray crystallography, NMR Spectroscopy, and electron microscopy. X-ray crystallography is one of the best methods because of the very high resolution that is a result of the wavelength, which is very close to that of the bonds between the atoms.
1. X-ray Crystallography
[edit | edit source]The first step in this method is to turn the protein into a crystal, which is done by adding certain salts that crystalize the macromolecule. Once it is in its crystal form, an X-ray beam is aimed at the protein and what happens to the light is observed. Some light will be absorbed, some will be scattered, and some will be diffracted. All of the rays are studied closely and their information, such as amplitude for example, give biochemists information about the atom in the molecule.

2. Nuclear Magnetic Resonance Spectroscopy
[edit | edit source]Another technique, called Nuclear Magnetic Resonance Spectroscopy, allows protein structure to be studied without being first converted into a crystal. It is one of the very few methods that allows the substance to be studied in solution, which is usually CDCl3. The two types of NMRs commonly used are proton NMR and carbon NMR, due to the magnetic resonance of the elements. For H-NMR, when a molecule is exposed to a magnetic and proton get excited and line up, four things can be observed that tell us about the environment of each identical group of protons. The chemical shift (how far downfield the signal is) tells what functional groups are surrounding the proton(s). The signal integration gives us a ratio that compares the amount of protons in each family. The splitting(amount of peaks in one signal, such as a doublet, triplet, etc) tells us how many neighboring hydrogens there are. And finally, the number of signals itself tells us how many different families of protons there are. C-NMR works the same way except for integration, which is not always clear as it is in H-NMR. So all this information is gathered for a molecule and its exact connectivity can usually be determined, even if it was an unknown molecule.

3. Electron Microscopy
[edit | edit source]The third method is electron microscopy, which uses uses an electron microscope that conducts an electron ray. The electron beams are aimed at the macromolecules and then impose an image of the molecule and its structure upon fluorescent or photographic film for us to see. Sometimes they are even sent directly to a screen, such as a computer screen. This image is a black a white picture but is still very useful in determining structure.
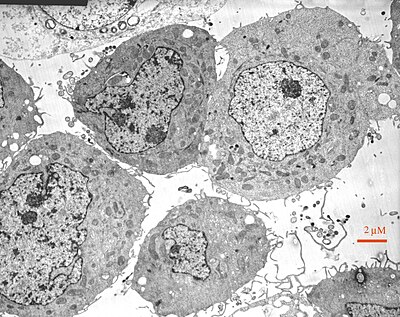
Sources
http://chem-guide.blogspot.com/2010/04/valence-shell-electron-pair-repulsion.html
http://www.rcsb.org/pdb/101/static101.do?p=education_discussion/Looking-at-Structures/methods.html
Biochemistry 6th Edition by Berg, Tymoczko, and Stryer
http://chemwiki.ucdavis.edu/Inorganic_Chemistry/Molecular_Geometry