User:Eyrunh
Ants
[edit | edit source]About an ant
[edit | edit source]Ants, sometimes referred to a as the power of nature, are remarkable insects. They evolved from wasp like ancestors about 100 million years ago and belong to the order Hymenoptera like wasps and bees[1]. Ants are highly socialize creatures, in fact, their whole existence depends on communication in the colony, which make them an almost unstoppable force. Most colonies consist of fertile females called queens, fertile short-lived males and sterile female which form the groups of workers or other specialized roles inside the colony. They thrive in most ecosystems and can be found almost all over the planet.

Colonies can be long lived with the queen sometimes living up to 30 years and the workers for 1-3 years [2]
As communication is essential for the colony survival, they have very elaborated ways to communicate. Ants communicate with each other mainly through sounds, touch and smell, that is, by the use of pheromones [3]. Ants perceive the smell through their antennae like most insects. The advantage of having two antennae is that the direction and intensity of the scents is easier to perceive. They use their scent in great quantity for food trails, that is, if an ant finds a bountiful food source he leaves a continuous scent of trail for other ants to follow later on and those ants add to the trail, resulting in a high intensity of the scent. When the food source is drained the ants stop leaving this scent and gradually, it diminishes [4]. These pheromone trails are not only used for food sources but also i.e. if an ant is crushed to death, it leaves a very strong alarm pheromone that send the ants into an attack frenzy and attracts more ants, they also use pheromones to pass information within the colony to know which task group they belong to and to identify kin and nest mates [1].These pheromones are produced mainly in the Dufour‘s gland (a gland that is characterizing for species of the Hymonoptera branch) [5] . For communication some ants also use sounds, which they mainly sense as vibration through their feet [1]
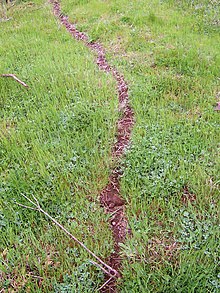
The Antennae
[edit | edit source]The antennae is a crucial sensory organ for the ant. Ants have two elbow like antennae and they are their primary olfactory sensor. They are well equipped with sensilla, a simple sensory receptor with small pores in it that capture odor volatiles [6]. As well as being used for odor sensing it also plays a role as a mechanoreceptor and is thought to play a major role in ant orientation by perceiving magnetic fields [7]. In the pedicel or the second segment of the antennae an organ called Johnston‘s organ is located. The Johnston‘s organ perceives movement in the flagellum (third part of the antennae) [8] and is also thought to play a major role in sensing magnetic fields [7]
Ant brain
[edit | edit source]The ant brain is mainly composed of antennal lobes, optic lobes, mushroom body calyx and protocerebral mass. The visual lobes vary between species, since some ant species use their eyes substantially (i.e. the Australian bulldog ant for hunting [9]), while for some species the small workers are blind. The olfactory lobes are big since almost their whole existence relies on their sense of smell. Ants are believed to have a relatively big brain for an insect [10]. The size of the ant brain varies between species and role in the colony with the queen having the largest brain.
Olfactory processing
[edit | edit source]As mentioned above ants heavily depend on chemical communication and these communications are essential to organize their social behaviour [1]. It is crucial for trail following behaviour, alertness, recruitment, reproductive state for the queen and for recognition of nestmates [11]. Thus the detection, processing and recognition over huge variety of chemical cues is important for the survival of an ant colony [12]. Like mention in the chapter about insect olfactory system (see [olfactory system]), the first stop for odor recognition is the sensillum on the antennae. From there, the odor molecule travels through one of the pores and land on a wax wall were they diffuse through. A pheromone binding protein arrives and ferries it across the sensillar lymph and binds to a receptor on the other site at the bottom of the olfactory receptor neurons (ORNs) This process is though not fully understood and is still under research [13]. The first relay station in the brain where the olfactory processing begins after the odor molecule has been ferried across is the antennal lobes (ALs) which receive information from the ORN. The ORN axons terminate in the olfactory glomeruli (cluster of nerve endings) within the AL. These glomeruli are considered to be the functional units in odor processing [14], but it has been shown that ants of the type C.florinadus contain high number of olfactory glomeruli (up to 464) in the AL which are supplied via seven distinct antennal sensory tracts (T). This vast number of glomeruli is almost 3 –times higher than in the honeybee [12]. Axons that express the same odorant receptor or have similar response profile end up in the same glomeruli. After some processing the information is transferred via projection neurons (PNs) to the mushrooms bodies for higher integration and to olfactory neuropils in the lateral protocerebral lobe (LPL) mostly in the lateral horn (LH). Up till now structural data on the detailed organization of the olfactory pathway is rather limited for ants [12].
Caste-and sex-specific differences in the morphology of the AL
[edit | edit source]The antennal lobe structures in social insects, like ants, show sex-or caste-specific organizations. One of the ant species that this has been verified is the carpenter ant Camponotus japonicus. For them there is a clear sex-dependent difference in number of glomeruli : females have over 430, while males have only about 215 including one macroglumerus [15]. Furthermore, there is a dimorphic difference between sexes of the carpenter ant in the antennae since the females have some type of sensillum that the male don‘t. But ants have different types of sensory neurons in some types of antennal sensilla, there have i.e. been identified alarm substance-receptive neurons in trichoid curvata sensilla of the black garden ant, a carbon-dioxide receptive neuron in an ampullaceal sensillum of the leaf-cutting ant, cuticular hydrocarbon-receptive neurons in basiconic sensilla of the carpenter ant and a cold-receptive neuron in coelconic sensillum of the leaf-cutting ant. The locations of different types of sensilla can be mapped to different regions of the glomerular clusters in the antennal lobe [15].

In the antennae are dorsal and ventral nerves. Axons of the antennal sensory neurons form bundles and enter the dorsal or ventral antennal nerves. Number of sensory neurons belonging to the dorsal nerve is much larger than that belonging to the ventral nerve. At the site of entry to the antennal lobe, afferents from the dorsal and the ventral nerve start to mix meaning the transition from a topographic organization of afferents to chemotopic organization. The first branch is divided into four sensory tracts (T) T1-T3 and T6. The second branch is divided into three sensory tracts T4, T5 and T7. Interestingly, the antennal lobes of workers and virgin females of the type Camponotus japonicus, all have these seven tracts while the antennal lobe in males of same species lack T6 and related glomeruli [15]. Additionally, in the glomeruli of workers and virgin females of this specie, sensory axons from either antennal nerve appears to converge densely while the axons terminal in glomeruli of male antennal lobe are not densely converged compared with those in workers or virgin females [15]. Differential labelling of two antennal nerves in the carpenter ant has revealed distinct glomerular clustering and a female-specific glomerular cluster in the antennal lobe. The male antennal lobe of the carpenter ant is completely missing T6 glomeruli, whereas workers and virgin females possess about 140 T6 glomeruli. The basiconic sensilla are lacking in the male antennae but exist in the antennae of workers and females indicating that the basiconic sensilla project into the T6 glomeruli. The numbers of sensory neurons housed by each basiconic sensillum is similiar to that of T6 glomeruli, indicating that sensory neurons that express single odorant receptor converge onto single glomeruli [16]. Sensory neurons expressing similar odorant receptors are mapped to closely appositioned glomeruli on the primary olfactory centre.
The basiconic sensilla respond to cuticular hydrocarbons and have been suggested to include function of nest-mate discrimination in the ant [17]. About 140 of T6 glomeruli might include a similar chemosensory function such as cuticular hydrocarbon receptive. The possibility that the basiconic sensilla are involved in detection of chemicals related to foraging, feeding and brood-caring is also possible but further investigation is needed on that matter [15]. Another interesting fact is that the T6 glomeruli does not contain serotonin-immunoreactive fibers while all other glomeruli do. The functional significance of the presence or absence of these fibers in ants is unclear, but it is likely that T6 glomeruli have functions of female specific tasks in the colony rather than sexual behaviour of the ant [15].
Another difference in the morphology of the brain between castes is the presence of two macroglomeruli assigned to the T3 glomeruli in the male antennal lobe. Such macroglomeruli are not observed on workers or virgin females but it is not clear whether these macroglomeruli are male-specific or sexually dimorphic. Similiar macroglomeruli have been observed in female cockroaches and moths. The location of one of these macroglomeruli is at the entrance of the antennal nerves in the antennal lobe and may have a female sex pheromone-recepetive function as macroglomeruli complex of many other male insect species located in same position have that function. The role of the other macroglomeruli in the male ant is still unclear [15].
Odor representation in the Antennal Lobe
[edit | edit source]Calcium-imaging in the AL have shown odor-induced activity of PNs. Furthermore; activation patterns in response to different odors have shown to be overlapping indicating that some glomeruli contribute to the activation pattern elicited by different odors and that odor specific activity patterns are conserved between individuals. In some cases, calcium signals from the PNs can be assigned to individual glomeruli but large activity regions indicate that odor used for stimulation is represented in several adjacent glomeruli rather than in isolated single glomeruli. Interestingly the activity regions covering the several glomeruli stays the same over large range of concentrations and is in most cases only slightly smaller at odor concentration level. In a response to nonsex pheromones, there is no difference in activity patterns in comparison to general odors [12].
Concentration threshold for odor detection is extremely low or 0.05 pg (equivalent to dilutions of 10-11). The threshold is similar for pheromones and general odors. The spatial activation pattern in response to the trail component is largely invariant over a large range of concentration (8-log units) and only at the lowest and highest concentration glomeruli disappeared or additional ones joined in. While in honeybees this range is limited. The reason for change in activity patterns at the level of AL is probably due to the fact that the receptive range of the ORNs is concentration dependent and broadens with increase intensity and can lead to different actitivity. In ants this could i.e. result in a well-defined behavioral sequence when approacing a pheromone source. The fact that the ants have no change in the spatial activation pattern over a such a large range in response to trail pheromones could be due to in sampling of chemical trail, antennation movements may cause large fluctuations at ORNs and therefore it may be important to maintain a constant spatial pattern to stabilize odor quality perception. The maintenance of constant quality perception and assessing odor intensity at the same time is important for trail –following behaviour. The activity pattern is the important parameter for assessing odor quality while the the peak activity if the PNs assess the odor intensity [12].
Higher-order olfactory centers
[edit | edit source]Most uniglomeral PNs send axons through the m-.APT (medial antenno-protocerebral tract) , have collateral projections to the mushroom-body calyces (MBCs) and terminate in the lateral horn (LH) of the protocerebrum [18] In the calyces of the mushroom body, the PNs synapse with Kenyon cells. The mushroom body is subdivided into lip, colar and basal ring, with different neurons terminating in each of them. Anatomical separation of input channels may not indicate separation of information if the third-order neurons sample across channels [19]. In contrast to the MBC the lateral horn of the protocerebrum in many insects appears to be a diffuse, aglomerular neruopil. PNs with a common neuroblast origin project to similar regions of the LH. Segregation of inputs in the LH is largely correlated with the input tract through which axon project, or by a distinction between food-derived and social or pheromonal stimuli. Little is known about olfactory information processing beyond the MB and LH [19].
Comparative coding
[edit | edit source]The output of the ORCs is a function of both the intensity and the identity of an olfactory stimulus. The circuitry of the AL needs to maintain sensitivity, prevent saturation, encode stimulus intensity, and maintain a consistent representation of stimulus across a range of concentration, as mentioned before. In order for that to work there needs to be a control of gain, that is, a conversion of signal strength between input and output to make efficient use of the dynamic range of PN output [19]. The synapse between PNs and ORC has a high probability of neurotransmitter release and the transformation between ORC input and PN output is highly nonlinear, amplifying weak and suppressing strong inputs. The input to the AL is typically a combination of weak activation of many ORC and a strong activation of few [20]. PN responses are equally likely across all possible firing rates due the nonlinear transformation which amplifies small differences between the weaker inputs. Global inhibition increases with total ORC input and because of that the representation of an olfactory stimulus is maintained by the relative activation of PNs across the AL over wide range of concentration[21]
Inhibition is crucial to neuronal circuits to prevent that under-stimulated units participate in the output. This mechanism is so called sharpening, meaning, that a stimulus is represented by the most strongly activated neurons, and overlap between codes for different stimuli is therefore reduced. On the other side there is also broadening, that is, network mechanisms broaden the molecular receptive range of PNs with respect to that of the ORCs. It can happen in two ways 1) the PN may respond to volatiles that do not activate cognate ORCs, or 2) the PN may respond to volatiles that do not activate cognate ORCs [19]. PNs respond to the rising phase of the ORC response but that response declines before the peak of the ORC activity. It is likely that the inhibitory network along with the properties of synapses in the AL, improves the ability to encode rapid spatio-temporal fluctuations of a plume of volatiles [19].
References
[edit | edit source]- ↑ a b c d Hölldobler, B., & Wilson, E. O. (1990). The ants. Harvard University Press.
- ↑ Keller, L. (1998). Queen lifespan and colony characteristics in ants and termites. Insectes Sociaux, 45(3), 235-246.
- ↑ Jackson, D. E., & Ratnieks, F. L. (2006). Communication in ants. Current biology, 16(15), R570-R574.
- ↑ Goss, S., Aron, S., Deneubourg, J. L., & Pasteels, J. M. (1989). Self-organized shortcuts in the Argentine ant. Naturwissenschaften, 76(12), 579-581.
- ↑ Mitra, A. (2013). Function of the Dufour’s gland in solitary and social Hymenoptera. Journal of Hymenoptera Research, 35, 33.
- ↑ Steinbrecht, R. A. (1996, January). Structure and function of insect olfactory sensilla. In Ciba Foundation Symposium 200-Olfaction in Mosquito-Host Interactions (pp. 158-183). John Wiley & Sons, Ltd.
- ↑ a b Vowles, D. M. (1954). The orientation of ants. Journal of Experimental Biology, 31(3), 341-355.
- ↑ Kamikouchi, A., Inagaki, H. K., Effertz, T., Hendrich, O., Fiala, A., Göpfert, M. C., & Ito, K. (2009). The neural basis of Drosophila gravity-sensing and hearing. Nature, 458(7235), 165.
- ↑ Eriksson, E. S. (1985). Attack behaviour and distance perception in the Australian bulldog ant Myrmecia nigriceps. Journal of Experimental biology, 119(1), 115-131.
- ↑ Gronenberg, W., & Hölldobler, B. (1999). Morphologic representation of visual and antennal information in the ant brain. Journal of Comparative Neurology, 412(2), 229-240.
- ↑ Hölldobler, B. (1995). The chemistry of social regulation: multicomponent signals in ant societies. Proceedings of the National Academy of Sciences, 92(1), 19-22.
- ↑ a b c d e Zube, C., Kleineidam, C. J., Kirschner, S., Neef, J., & Rössler, W. (2008). Organization of the olfactory pathway and odor processing in the antennal lobe of the ant Camponotus floridanus. Journal of Comparative Neurology, 506(3), 425-441.
- ↑ Renou, M. (2014). Pheromones and general odor perception in insects. Neurobiology of chemical communication, 23.
- ↑ Hildebrand, J. G., & Shepherd, G. M. (1997). Mechanisms of olfactory discrimination: converging evidence for common principles across phyla. Annual review of neuroscience, 20(1), 595-631.
- ↑ a b c d e f g Nakanishi, A., Nishino, H., Watanabe, H., Yokohari, F., & Nishikawa, M. (2010). Sex‐specific antennal sensory system in the ant Camponotus japonicus: Glomerular organizations of antennal lobes. Journal of Comparative Neurology, 518(12), 2186-2201.
- ↑ Gao, Q., Yuan, B., & Chess, A. (2000). Convergent projections of Drosophila olfactory neurons to specific glomeruli in the antennal lobe. Nature neuroscience, 3(8), 780.
- ↑ Ozaki, M., Wada-Katsumata, A., Fujikawa, K., Iwasaki, M., Yokohari, F., Satoji, Y., ... & Yamaoka, R. (2005). Ant nestmate and non-nestmate discrimination by a chemosensory sensillum. Science, 309(5732), 311-314.
- ↑ Homberg, U., Montague, R. A., & Hildebrand, J. G. (1988). Anatomy of antenno-cerebral pathways in the brain of the sphinx moth Manduca sexta. Cell and tissue research, 254(2), 255-281.
- ↑ a b c d e Martin, J. P., Beyerlein, A., Dacks, A. M., Reisenman, C. E., Riffell, J. A., Lei, H., & Hildebrand, J. G. (2011). The neurobiology of insect olfaction: sensory processing in a comparative context. Progress in neurobiology, 95(3), 427-447.
- ↑ Bhandawat, V., Olsen, S. R., Gouwens, N. W., Schlief, M. L., & Wilson, R. I. (2007). Sensory processing in the Drosophila antennal lobe increases the reliability and separability of ensemble odor representations. Nature neuroscience, 10(11), 1474.
- ↑ Sachse, S., & Galizia, C. G. (2003). The coding of odour‐intensity in the honeybee antennal lobe: local computation optimizes odour representation. European journal of neuroscience, 18(8), 2119-2132.