Sensory Systems/Arthropods/Mantis Shrimp
Visual system of the Mantis shrimp
[edit | edit source]
Introduction
[edit | edit source]Mantis shrimps or Stomatopods are a family of crustaceans that are usually between 10 and 20 cm in length. They can be brightly colored and live in shallow waters in tropical or subtropical oceans. A big part of the Stomatopod's thorax is covered with a resilient carapace with the head in the front and the two eyeballs sticking out on a pair of stalks. They carry multiple pairs of limbs where the second pair is noticeably larger and known for excellent punching abilities. What they are probably most famous for is though their complex visual system. [1]
A unique visual system
[edit | edit source]Mantis shrimps have one of the most complex visual system discovered in animals. Instead of using 2-4 photoreceptors types for color vision like most other species, they use 12! In addition, they have 4-7 receptor types (depending on the species) that are sensitive for linear and circular polarized light. [2] This has made people wonder how the Mantis shrimps see the world and if they have a 12-dimensional color space compared to our 3-dimensional.
Scientists have assumed that having so many types of photoreceptors would make the Mantis shrimp able to distinguish colors only a few nano-meters apart if it made analog comparisons between spectral sensitivities. On the contrary, a recent study showed that they have trouble distinguishing colors less than 25 nm apart which is approximately the distance between the sensitivity peaks of different photoreceptors. This suggests that the animals don't process visual information by comparing input from different photoreceptors like humans do but rather detect which receptor gives the strongest signal. [2] This would mean that the Mantis shrimps do not have a 12-dimensional continuous color space but rather a discrete color space with 12 color bins. The advantage of this system is that it allows the animals to determine colors very fast and reliably without the delay that occurs in a multidimensional color space. The neural processing of the system is though still to be determined. [2]
Anatomy of the eye
[edit | edit source]The eye of Mantis shrimps is a compound eye made up of optical units called ommatidia. An ommatidia has a lens that is covered with a cornea and behind the lens there is a light guide, called rhabdom. Surrounding the rhabdom are photoreceptors that can be sensitive to ultraviolet light or light in the human visible range. [3]The eyes are usually elliptical and are divided in morphologically different zones. [4] Each eye is divided horizontally in three regions, the dorsal hemisphere, the mid-band and the ventral hemisphere which all explore the space. [2] [4] It is believed that the two hemispheres allow the animal to have stereoscopic vision on each eye. The ommatidia are arranged in rows where each row has the same morphology. [4]
The ommatidia in the hemispheres of the eye are similar to the ommatidia found in other crustaceans.[5]The mid-band contains larger, specialized ommatidia with photoreceptors that are responsible for most of the spectral diversity. [6] Having the mid-band horizontally between the hemispheres makes the animal able to keep objects anywhere on the horizon within the focal area without much horizontal saccadic eye movements. In fact, most saccadic movements are vertical. [4] Rows 1 through 4 of the mid-band are involved with color vision while rows 5 and 6 detect linear and circular polarized light. In rows 1 to 4 there are 12 different types of cells, each sensitive to a different wavelength of light. Additionally, there are 4 cell types sensitive to ultraviolet light distally in the first four rows. [2] [4]
Each rhabdom has an individual optic system which results in a high number of optical units. This way, all photoreceptors in an ommatidium can view the same field for simultaneous analysis of different properties. Two separate regions in the same eye can even view the same field which makes the system flexible and increases the possibilities of parallel processing. The disadvantage is an eye with low spatial resolution compared to its size. Ommatidia in each hemisphere of the eye are able to view the same field which makes each eye stereoscopic. [5]
Each eye is seated on a stalk and can move relatively freely in all axes thanks to six groups of muscles. In addition, each eye can move independently of the other. The independent moving of each eye makes it hard to make use of binocular stereopsis. Instead, they probably use the overlapping of the view of the two hemispheres of each eye to estimate distance. When the stalks of the eyes move, distant objects move more slowly than close objects which adds to their depth perception. [5] The top part of the ommatidium in the hemispheres is composed of a cornea above a crystalline cone. This part is dedicated to the optics and focuses the incoming light onto the photosensitive rhabdom below. The rhabdom is composed of eight receptor cells, the R8 cell at the top and cells R1-7 surrounding the rhabdom below. These cells form a light guide. The R8 cell is only sensitive to ultraviolet light while cells R1-7 are sensitive to wavelengths around 500 nm. The R8 cells are not sensitive to polarization but cells R1-7 have two types of receptors that are sensitive to polarization orthogonal to each other. [5]
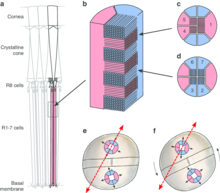
The ommatidia in the mid-band of the eye are different from those in the hemispheres and the mid-band contains three types of ommatidia. The first type of ommatidia are in the two most ventral rows and sense polarized light. The R8 cells of each of the two rows sense polarization planes orthogonal to each other. The R1-7 cells sense orthogonal wavelengths around 500 nm using two types of receptors. The R8 receptors also convert circular polarized light to linear polarized light that is then sensed by the R1-7 receptors.
The second type of ommatidia are in two of the four most dorsal rows. The R1-7 cells are split in two layers so incoming light first goes through the ultraviolet sensitive R8 part, then the distal part of R1-7 and finally the proximal part. Each layer absorbs certain wavelengths before the light reaches the layers below, together creating narrow-band photoreceptors.
The third type of ommatidia are in the two remaining rows. They contain colored photo-stable filters between the receptor layers so incoming light is filtered by these filters as well as the absorption of the receptors.
The second and third type of ommatidia are insensitive to polarization. The four rows of the second and third type of ommatidia, have two types of receptor layers each. These total of eight receptor types have different visual pigments so together they span the spectrum of around 400-700 nm. Adding the ultraviolet receptors and the polarization sensitivity, gives around 16-21 receptor classes, depending on the species. [5][2]
Ultraviolet vision
[edit | edit source]Unlike humans, Mantis shrimps are able to detect ultraviolet light. The ultraviolet photoreceptors are evenly spaced in the eye which suggests that the ultraviolet vision in Mantis shrimps is a part of their color vision system with sensitivity in the range of 300-700 nm wavelengths, compared to 400-700 nm in humans. The ultraviolet sensitivities are too narrow to result only from visual- pigment absorption which made scientists believe that they were tuned with ultraviolet filters in the photoreceptors. [7]
A recent experiment found four types of ultraviolet absorbing MAA (mycosporine-like amino acids) in the mid-band that are sensitive to ultraviolet light. These pigments work as either short- or long-pass ultraviolet filters that act on the same visual pigment in the retina, multiplying the sensitivity of the ultraviolet spectrum. This way, they can generate six types of ultraviolet receptors. [6] [8]
In deep waters, fluorescence can contribute more to color than on land because of its contrast to the surrounding blue color. Many sea organisms have fluorescent coloration, one of them is the Mantis shrimp. The Mantis shrimp species Lysiosquillina glabriuscula has fluorescent markings on its antennal scales and carapace. The fluorescence is estimated to give rise to 7-10% of the total photons from the markings in the depth range inhabited by the animal. When looked at from the L. glabriuscula's perspective, the fluorescence is of more importance because of their special visual system and accounts for up to 30% of the total number of photons. The fluorescence makes the animal able to enhance its color signal underwater where shorter wavelengths don't arrive. [9]
Polarisation vision
[edit | edit source]Polarisation can be described with the Stokes' parameters,
with as the intensity and standing for horizontal, vertical, diagonal, anti-diagonal, right-hand circular and left-hand circular, respectively. S0 is the total intensity which does not affect the polarization. Polarized light is common in nature, especially reflected light and arthropods as well as crustaceans are sensitive to linearly polarized light. It can for example give information about the texture and orientation of an object. A single linear polarization component provides more contrast, especially in turbulent water, while more linear components probably influence orientation, navigation, prey detection, predator avoidance, and intra-species signaling.
Optimal polarization vision is simultaneous sensitivity to all six linear and circular polarized components and the Gonodactylidae family of the Mantis shrimps is the first organism discovered that possesses this ability. The dorsal and ventral hemispheres in their eye sense linear polarization, rotated 45 deg from each other. What makes the Gonodactylidae special is though their sensitivity for circular polarization in two rows of the mid-band which makes it possible for them to measure all six Stokes' parameters. In addition to these anatomical features, it has the neuronal features for measuring the Stokes' parameters. [3] [10] [5]
Filtered vision
[edit | edit source]Different Mantis shrimp species live at various depths. Animals that live in shallow waters are exposed to illumination over a much broader spectrum than animals in deeper waters. Experiments on the Mantis shrimp species Haptosquilla trispinosa revealed that they use colored filters in front of their photoreceptors to tune the spectral sensitivity. In animals that live in shallow waters, the filters are used on most of the visible spectrum. Meanwhile, those that live in deeper waters have their filters shifted to transmit shorter wavelengths (green-blue light) as longer wavelengths are attenuated by the water. This makes them able to distinguish smaller differences of the short wavelength light in the ocean. [11]
Visual processing
[edit | edit source]The visual processing in Mantis shrimps is different from humans and may be compared to artificial systems as they use serial and parallel processing. Mantis shrimps must move their eyes to collect some types of visual information from the environment, unlike most other animals. This lies in the fact that the most important region for visual analysis is in the narrow mid-band which can only scan a slice of the visual space. Mantis shrimps solve this problem by moving the eye slowly up and down and thus getting information about color, polarization and ultraviolet intensity for the whole visual field.
A lot of the visual processing in Mantis shrimps takes place within the eye and even in single photoreceptors. This decreases the amount of data needed to deliver information to higher areas. From the retina, it appears that information is sent via multiple parallel streams into the central nervous system which makes it able to minimize processing at higher levels. Another advantage of Mantis shrimps' splitting of the visual spectrum into discrete channels is its color constancy. Visual systems that have few receptors with a broad wavelength spectrum, can adapt strongly to wavelengths that are far from their peak sensitivity wavelength which makes it difficult to recognize colors in different environments, such as underwater. [5]
Benefits from a developed visual system
[edit | edit source]Mantis shrimp lives involve incredibly fast movements while attacking prey which makes it impor- tant to have fast processing of visual information. [2] Mantis shrimps are known to attack, not only to hunt prey but also to fight members of the same species. This is believed to have evolved their signaling behaviour that involves polarised light and color. They use their color in signaling to a greater extent than other crustaceans and it is believed that their special visual system with high color constancy makes that possible. [5]
Inspiring technology
[edit | edit source]Many features of the visual system of Mantis shrimps could influence the development of artificial optical systems. When designing optical systems where color constancy is important, the Mantis shrimps' visual system can be used as a model where the narrow spectral channels increase the accuracy. Contrary to current optical sensor properties, motion is essential to the Mantis shrimp's vision. This opens up the idea of integrating the possibility of motion into optical sensors. [5] Mantis shrimps' eye design is a good model for visual electronics as it is able to do analysis within individual units. Its visual processing in the eye before information gets passed on to higher centers is also an inspiration for efficient, low-power artificial optical systems. Processing data at the sensor level can reduce the bandwidth and the power needed. Their polarization sensitivity has also inspired scientists in the development of polarization sensors. In fact, Mantis shrimps' alignment of polarization sensitive ommatidia has been replicated with aluminum nanowires functioning as linear polarization filters on top of photo-diodes to create a CMOS imager. This real-time polarization imaging has enabled early diagnosis of cancerous tissue that has not been possible before and has many potential future applications. [12]
References
[edit | edit source]- ↑ Ross Piper, Extraordinary Animals: An Encyclopedia of Curious and Unusual Animals, Green- wood Press, 2007.
- ↑ a b c d e f g Hanne H. Thoen et al, A Dierent Form of Color Vision in Mantis Shrimp, Science 343: 411- 413, 2014.
- ↑ a b Kleinlogel S, White AG, The Secret World of Shrimps: Polarisation Vision at Its Best, PLoS ONE 3(5): e2190, 2008.
- ↑ a b c d e David Cowles, Jaclyn R. Van Dolson, Lisa R. Hainey, Dallas M. Dick, The use of dierent eye regions in the mantis shrimp Hemisquilla californiensis Stephenson, 1967 (Crustacea: Stom- atopoda) for detecting objects, Journal of Experimental Marine Biology and Ecology 330 (2): 528534, 2006.
- ↑ a b c d e f g h i Thomas W. Cronin, Justin Marshall, Parallel processing and image analysis in the eyes of mantis shrimps, The Biological Bulletin 200 (2): 177183, 2001.
- ↑ a b Michael Bok, Megan Porter, Allen Place, Thomas Cronin, Biological Sunscreens Tune Polychro- matic Ultraviolet Vision in Mantis Shrimp, Current Biology 24 (14): 163642, 2014.
- ↑ Justin Marshall, Johannes Oberwinkler, Ultraviolet vision: the colourful world of the mantis shrimp, Nature 401 (6756): 873874, 1999.
- ↑ Ellis R. Loew, Vision: Two Plus Four Equals Six, Current Biology 24 (16): 753-755, 2014.
- ↑ C. H. Mazel, T. W. Cronin, R. L. Caldwell, N. J. Marshall, Fluorescent enhancement of sig- naling in a mantis shrimp, Science 303 (5654): 51, 2004.
- ↑ Tsyr-Huei Chiou et el, Circular polarization vision in a stomatopod crustacean, Current Biology 18 (6): 42934, 2008.
- ↑ Thomas W. Cronin, Roy L. Caldwell, Justin Marshall, Tunable colour vision in a mantis shrimp, Nature 411, 547, 2001.
- ↑ T. York et al, Bioinspired polarization imaging sensors: from circuits and optics to signal processing algorithms and biomedical applications, Proceedings of the IEEE 102 (10): 14501469, 2014.