Pulsars and neutron stars/History of telescopes and instrumentation
Telescopes
[edit | edit source]The first pulsar was serendipitously discovered using a radio telescope array (known as the 4-acre array) that was being used to search for quasars. Every day, the telescope signal was recorded on several rolls of paper, which were inspected by eye. Since then, technology has progressed; nowadays supercomputers process pulsar observations coming from some of the largest and most advanced telescopes on Earth and in space.
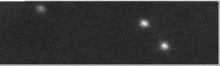
The Crab pulsar is one of the best studied pulsars. It was first detected as a pulsing radio source using the 300-ft Green Bank telescope (Staelin & Reifenstein 1968). Optical pulses were detected a year later by Cocke, Disney & Taylor (1969). Not long after, the first X-ray pulsations to be detected in the Crab nebula were reported by Fritz et al. (1969). Vasseur et al. (1970) used a balloon experiment and tentatively detected pulsed gamma ray emission from the Crab pulsar. TeV gamma rays from the Crab nebula and pulsar were observed using a Cerenkov imaging method (Weekes et al. 1989). The Crab pulsar has also been studied using gravitational wave telescopes (Abbott et al. 2007), with cosmic ray detectors (Wdowczyk & Wolfendale 1983) and as a possible neutrino source (Aartsen et al., 2015).

The array that first discovered pulsars is now no longer in use. However, the Lovell telescope at Jodrell Bank Observatory (1957) and the Parkes (1962), Arecibo (early 1960s) and Nançay (1965) telescopes, all constructed prior to the discovery of the first pulsar, are still active in pulsar research, which now involves most of the major radio telescopes around the world. Relatively new radio telescopes include low-frequency telescopes such as LOFAR (e.g., Fender 2007), the GMRT, the Murchison Widefield Array (see e.g, Tremblay et al. 2015) and the Long Wavelength Array (Stovall et al. 2015). At higher frequencies the Molonglo radio telescope is currently being refurbished and the Sardinian radio telescope first observed a pulsar around 2011. The world’s largest single-dish radio telescope (the Five Hundred Meter Aperture Spherical Telescope, FAST) is currently being assembled in China (Peng et al. 2000). It is expected that this telescope will commence observations during 2016.
To date, RadioAstron is the only space-based radio telescope that has been involved in pulsar research (Rudnitskiy, Mikhail & Vladimir 2014), but many other space-based telescopes are observing pulsars in other wavebands. For instance, the Hubble Space telescope has observed pulsars in the optical and ultraviolet (Percival et al. 1993). X-ray telescopes (see e.g., An et al. 2014 for NuSTAR) and gamma-ray telescopes (e.g., Thompson et al. 1994) have also been used to detect and observe pulsars. High-energy emission can also be detected using ground-based detectors such as the VERITAS array (Aliu et al. 2015).
Telescopes are also being used in combination. For instance Galt et al. (1970) observed PSR B0329+54 using an interferometer. Now, pulsar science is a key goal of Very-long-baseline interferometry (VLBI) networks. Kramer & Stappers (2010) describe the Large European Array for Pulsars (LEAP) project in which the data from Europe's largest telescopes is being combined. A major VLBA pulsar astrometry program, PSRpi, was started by Deller et al. (2011). Not only are telescopes of the same type being used together, but now astronomers carry out coordinated X-ray, ultraviolet, optical and radio observations (see e.g., Bogdanov et al. 2015).
A new era in pulsar research using radio telescopes will soon begin with the Square Kilometre Array (SKA; first mentioned by Wilkinson 1991) and its precursor telescopes such as MeerKAT and ASKAP. Bell (2000) and Cordes et al. (2004) provided early reports on how the SKA and other new facilities are expected to study pulsars. More recently, a large number of papers have been written on the role of the SKA for pulsar science, as summarised by Kramer & Stappers (2015). Phase 1 of the SKA is now in preparation. A low-frequency part of the telescope will be built in Western Australia using aperture array technology (aperture arrays have been described by van Bemmel et al. 2012). Higher-frequency observations will be carried out using an interferometer dish array in Southern Africa.
Receiver and backend instrumentation
[edit | edit source]It is remarkable how many of the old, single dish radio telescopes are still being used for cutting-edge pulsar research. This is because the observing capabilities at these telescopes have been repeatedly upgraded. An early review of pulsar instrumentation was presented by Rayner & Witham (1973) who described the status (at the time) of computer hardware and software for on-line pulsar searching. More updates to the hardware and software systems used were required after the discovery of the first millisecond pulsar in 1982 (Backer et al. 1982). Pulsar signals are affected by the propagation of the signal through the interstellar medium. In order to counteract the effect of the pulse dispersion it is necessary to divide the incoming data into a large number of frequency channels. A method that can, in principle, completely remove the effect of dispersion is known as "coherent dedispersion", but that method requires fast sampling of the incoming data and sufficient computing power to process the data. Hankins, Stinebring & Rawley (1987) demonstrated a real-time coherent dedispersion system that could process a bandwidth of 2MHz. The design of PuMa, a baseband recoding and digital filterbank system, was reported by Voute et al. (2002). This system could process a bandwidth of up to 20 MHz in baseband mode and 80 MHz as a digital filterbank. A wide band pulsar machine for Westerbork (PuMa-II) was described by Karappusamy, Stappers & van Straten (2008) and could process a bandwidth of 160MHz. Modern coherent dedispersion systems can process bandwidths of many GHz.
The backend developments have, in part, been driven by new receiver systems. Single-pixel radio receivers have become cooler and able to receive wider and wider bands. However, as telescopes grew larger larger their beams became narrower and hence any given survey of the sky would take longer to complete. In 1996 the Parkes telescope was upgraded with a 13 beam multibeam receiver (Staveley-Smith et al. 1996). Multibeam receivers are now in use at Parkes and Arecibo and a 19-beam system will soon be installed on the FAST telescope. In future it is likely that pulsar surveys will be carried out using focal plane arrays (see Verheijen et al. 2008 and Johnston et al. 2008).
The amount of data produced during a pulsar observation has always been large, requiring the development of new methods for storage and archiving. There is still no agreed-upon standard within the pulsar community for pulsar data sets. Matsakis, Josties & Foster (1996) first called for an international pulsar data archive and suggested the use of FITS for data storage, which was followed up by van Straten et al. (2010) who presented the PSRFITS file format. However, Lorimer et al. (1998) presented a different method for storing pulsar data. A large data archive of Parkes pulsar data (stored in PSRFITS format) was described by Hobbs et al. (2011).
Radio frequency interference (RFI) is becoming a larger and larger problem for pulsar observations. Bell et al. (2001) presented baseband data for testing interference mitigation algorithms. There is a need for significantly more research in this area in the near future.