High School Earth Science/Measuring and Predicting Earthquakes
Seismograms tell seismologists how strong an earthquake is and how far away it is. At least three seismograms must be used to calculate where the epicenter is located. Over the past century, scientists have developed several ways of measuring earthquake intensity. The currently accepted method is the moment magnitude scale, which measures the total amount of energy released by the earthquake. At this time, seismologists have not found a reliable method for predicting earthquakes.
Lesson Objectives
[edit | edit source]- List the different types of seismic waves, their different properties and describe how seismologists can use them to learn about earthquakes and the Earth's interior.
- Describe how to find an earthquake epicenter.
- Describe the different earthquake magnitude scales and what the numbers for moment magnitude mean.
- Describe how earthquakes are predicted and why the field of earthquake prediction has had little success.
Measuring Magnitude
[edit | edit source]A seismometer is a machine that records seismic waves. In the past, all seismometers were seismographs because they produced a graph-like representation of the seismic waves they received. The paper record is called a seismogram. Modern seismometers record ground motions using electronic motion detectors. The data are then kept digitally on a computer.
Seismographs have a pen suspended from a stationary frame, while a drum of paper rotates beneath it. The pen is weighted so that it is suspended and not attached to the ground. The drum is attached to the ground. As the earth shakes in an earthquake, the pen remains stationary but the drum moves beneath it. This creates the squiggly lines that make up a seismogram (Figure 7.39).

Seismograms contain information on how strong an earthquake was, how long it lasted, and how far away it was. The wiggly lines that are produced in a seismogram clearly show the different arrival times of P- and S-waves (Figure 7.40). As with words on a page, the seismogram record goes from left to right. First, there is a flat line, where there was no ground shaking. The first waves to be recorded by the seismogram are P-waves since they are the fastest. S-waves come in next and are usually larger than P-waves. The surface waves arrive just after the S-waves. If the earthquake has a shallow focus, the surface waves will be the largest ones recorded.

If a seismogram has recorded P-waves and surface waves, but not S-waves, the seismograph was on the other side of the planet from the earthquake. Scientists know that the earth's outer core is liquid because S-waves cannot travel through liquid. The liquid outer core creates an S-wave shadow zone on the opposite side of the planet from the earthquake's focus where no S-waves reach. The amplitude (height) of the waves can be used to determine the magnitude of the earthquake. How magnitude is calculated will be discussed in a later section.
Finding the Epicenter
[edit | edit source]A single seismogram can tell a seismologist how far away the earthquake was but it does not provide the seismologist with enough information to locate the exact epicenter. For that, the seismologist needs at least three seismograms. Determining distance to an earthquake epicenter depends on the fact that different seismic waves travel at different speeds. P-waves always arrive at a seismometer first, but the amount of time it takes for the S-waves to arrive after the P-wave indicates distance to the epicenter. If the epicenter is near the seismometer, the P-waves, S-waves and surface waves will all arrive in rapid succession. If the epicenter is further away, the S-waves will lag further behind. In other words, the longer it is between the arrival of the P-wave and S-wave from an earthquake, the farther the epicenter is from the seismometer.
After many years of study, geologists know the speed at which the different types of waves travel through various earth materials. Based on the difference in the arrival times of the first P wave and the first S wave, seismologists determine the distance between the epicenter and a seismometer. Once the distance to the epicenter is known, scientists can identify each point that is that distance away. Let's say that they know that an earthquake's epicenter is 50 kilometers from Kansas City. When each point that is that distance away from Kansas City is marked, the marks create a circle. This circle can be drawn with a compass.
To locate the earthquake epicenter, seismologists must have data from at least three seismometers. A circle drawn at the correct distance to the epicenter from a second seismometer will intercept the first circle in two places. A third circle showing the distance to the epicenter from a third seismometer will intercept the other two circles at a single point. This point is the earthquake epicenter (Figure 7.41). While this method was extremely useful for locating epicenters for decades, the technique has been replaced by digital calculations.

Earthquake Intensity
[edit | edit source]People have always tried to quantify the size of and damage done by earthquakes. Early in the 20th century, earthquakes could only be described in terms of what nearby residents felt and the damage that was done to nearby structures. This was called the Mercalli Intensity Scale and was developed in 1902 by the Italian seismologist Giuseppe Mercalli. The Mercalli Scale is sometimes used today in conjunction with the more modern intensity scales described below.
Table 7.1 shows an abbreviated description of the twelve Mercalli intensity levels:
Number | Description |
---|---|
I | Not felt except by a very few under especially favorable conditions. |
II | Felt only by a few persons at rest, especially on upper floors of buildings. |
III | Felt noticeably by people indoors, especially on upper floors of buildings. Many people don't know it's an earthquake. Standing automobiles may rock lightly. Vibrations similar to the passing of a truck. Duration estimated. |
IV | Felt indoors by many, outdoors by few during the day. At night, some awakened. Dishes, windows, doors disturbed; walls make cracking sound. Sensation like heavy truck striking building. Standing cars rocked noticeably. |
V | Felt by nearly everyone; many awakened. Some dishes, windows broken. Unstable objects overturned. Pendulum clocks may stop. |
VI | Felt by all, many frightened. Some heavy furniture moved; a little fallen plaster. Damage slight. |
VII | Damage negligible in buildings of good design and construction; slight to moderate in well-built ordinary structures; considerable damage in poorly built or designed structures; some chimneys broken. |
VIII | Damage slight in specially designed structures; considerable damage in ordinary substantial buildings with partial collapse. Damage great in poorly built structures. Fallen chimneys, factory stacks, columns, monuments, walls. Heavy furniture overturned. |
IX | Damage considerable in specially designed structures; well-designed frame structures thrown out of plumb. Damage great in
substantial buildings, with partial collapse. Buildings shifted off foundations. |
X | Some well-built wooden structures destroyed; most masonry and frame structures destroyed with foundations. Rails bent. |
XI | Few if any structures still standing. Bridges destroyed. Rails bent greatly. |
XII | Damage total. Lines of sight and level distorted. Objects thrown into air. |
There were many problems with the Mercalli scale. What people feel and see in an earthquake is affected by how far they are from the earthquake's focus, the type of rock that lies beneath them, the construction type of the nearby buildings, and many other factors. Different observers will also perceive the experience differently. For example, one might exaggerate while the other downplays the damage done. With the Mercalli scale, comparisons between earthquakes are difficult to make.
To address these problems, in 1935 Charles Richter developed his Richter magnitude scale. The Richter scale measures the magnitude of the largest jolt of energy released in an earthquake. Because Richter's scale is logarithmic, the amplitude of the largest wave increases 10 times from one integer to the next. For example, the amplitude of the largest seismic wave of a magnitude 5 quake is 10 times that of a magnitude 4 quake and 100 times that of a magnitude 3 quake. One integer increase in magnitude roughly correlates with a 30-fold increase in the amount of energy released. A difference of two integers on the Richter scale equals a 1,000-fold increase in released energy.
Seismologists recognize that the Richter scale has limitations, since it measures the height of the greatest earthquake wave. A single sharp jolt will measure higher on the Richter scale than a very long intense earthquake that releases more energy. In other words, earthquakes that release more energy are likely to do more damage than those that are short, but have a larger single jolt. Using the Richter scale, a high magnitude may not necessarily reflect the amount of damage caused.
The moment magnitude scale is the current method of measuring earthquake magnitudes. This method measures the total energy released by an earthquake and so more accurately reflects its magnitude. Moment magnitude is calculated from the area of the fault that is ruptured and the distance the earth moved along the fault. Like the Richter scale, the moment magnitude scale is logarithmic. An increase in one integer means that 30 times more energy was released, while two integers means that 1,000 times the energy was released released. The Richter and moment magnitude scales often give very similar measurements.
In a single year, more than 900,000 earthquakes are recorded. 150,000 of them are strong enough to be felt. About 18 per year are major, with a Richter magnitude of 7.0 to 7.9. Each year, on average, one earthquake with a magnitude of 8 to 8.9 strikes. Remember that many of these earthquakes occur deep in the crust and out in the oceans and do not cause much or any damage on land.
Earthquakes with a magnitude in the 9 range are rare. The United States Geological Survey lists six such earthquakes on the moment magnitude scale in historic times (see Figure 7.42 and Table 7.2). All but one of them, the Great Indian Ocean Earthquake of 2004, occurred somewhere around the Pacific Ring of Fire.

Location | Year | Magnitude |
---|---|---|
Chile | 1960 | 9.5 |
Prince William Sound, Alaska | 1964 | 9.2 |
Great Indian Ocean Earthquake | 2004 | 9.1 |
Kamchatka, Alaska | 1952 | 9.0 |
Africa, Peru (now Chile) | 1868 | 9.0 |
Cascadia Subduction Zone | 1700 | 9.0 |
Earthquake Prediction
[edit | edit source]To be valuable, an earthquake prediction must be accurate. A good predication would anticipate the date, location, and magnitude of the earthquake. The prediction would need to be accurate so that authorities could convince people to evacuate. An unnecessary evacuation would be very expensive and would decrease the credibility of authorities who might need to evacuate the region at a later time. Unfortunately, accurate predictions like these are not likely to be common for a long time.
The easiest thing to predict is where an earthquake will occur (Figure 7.43). Because nearly all earthquakes take place at plate boundaries, and because earthquakes tend to happen where they’ve occurred before, scientists know which locations are likely to have earthquakes. This information is useful to communities because those that are earthquake-prone can prepare for the event. For example, these communities can implement building codes to make structures earthquake safe. The added work and expense can be avoided in areas that are not at risk.
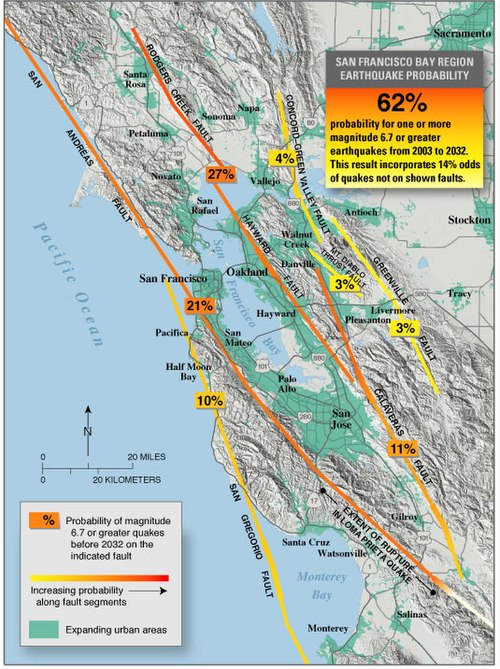
Predicting when an earthquake will occur is much more difficult. Scientists can get a general idea by looking at the historical and geological records of earthquakes in an area. If stress on a fault builds up at the same rate over time, then earthquakes should occur at regular intervals. While this is true, there is a large margin of error in these predictions. Using this method, scientists cannot even be accurate to within a few years, and evacuation is not practical.
Seismologists have also used the seismic gap theory for long-term earthquake prediction. In this theory, scientists assume that, on average, all of the rocks on the same side of a fault move at the same rate. For example, they say that rocks on the North American plate side of the San Andreas Fault in California move at the same speed over time. While this may be true, the frequency and magnitude of earthquakes along the fault is not the same: there are more quakes in the northern and southern sections, but a relatively inactive zone in the center.
Seismologists attempted to use the seismic gap theory to predict an earthquake in a seismic gap. Around Parkfield, California earthquakes occur regularly: an earthquake of magnitude 6.0 or higher occurs about every 22 years. Using this information, seismologists predicted that a magnitude 6 or greater earthquake would strike the region in 1993. In the mid-1980s, seismologists with the United States Geological Survey set up an enormous number of instruments along the Parkfield section of the San Andreas to monitor the expected earthquake. While they were right that an earthquake was due in that segment of the fault, they were quite far off in predicting the earthquake’s timing. A magnitude 6.0 quake did not strike Parkfield until 2004, 11 years late.
Scientists have recognized some indicators that allow them to recognize that a large earthquake is likely. Large earthquakes are often preceded by small tremors, called foreshocks, that occur between a few seconds and a few weeks before a major quake. However, many earthquakes are not preceded by foreshocks and clusters of small earthquakes are not necessarily followed by a large earthquake.
Large earthquakes are also often preceded by the tilting of the ground surface, which is caused by the buildup of stress in the rocks. Seismologists measure the ground tilt and use the changes to predict an impending earthquake. While this technique has been somewhat successful, it has also been a part of predictions of earthquakes that never came and has failed to predict some that did. Water levels in wells fluctuate as water moves into or out of fractures before an earthquake. This information can also be used as a possible, but uncertain, predictor of large earthquakes.
The most successful earthquake prediction was on February 4, 1975. At the recommendation of Chinese seismologists, officials evacuated many of the residents of the Manchurian province of Liaoning. Although the region was not prone to earthquakes, the seismologists made their prediction because the area experienced about 400 small foreshocks over a few days. The night of the evacuation an earthquake of magnitude 7.3 struck the town and only a few hundred people died. An estimated 150,000 people may have been saved. However, a little more than a year later, Chinese seismologists failed to predict the Tangshan earthquake, which killed more than 250,000 people. One month after that, Chinese officials evacuated residents of the Guandong Province for an earthquake that never came.
There is value in predicting the arrival of seismic waves from an earthquake that is already taking place. Seismometers can detect P-waves a few seconds before more damaging S-waves and surface waves arrive. Although a few seconds is not much, a coordinated computerized system can use that time to shut down gas mains and high tension electrical transmission lines, and initiate protective measures in chemical plants, nuclear power plants, mass transit systems, airports and on roadways.
Folklore tells of animals behaving erratically just before an earthquake. Mostly these anecdotes are told after the earthquake, when people remember back to the time before the shaking began. Memories are notoriously faulty. However, Chinese scientists actively study the behavior of animals before earthquakes to see if there is something to the anecdotes.
One interesting tale involves the number of animals killed in the 2004 Boxing Day Tsunami, which appeared to be surprisingly low. Reports abound suggesting that the animals had a "sixth sense" that warned them of the danger. In Sri Lanka's Yala National Park, for example, about 60 tourists and park employees drowned but few large animals. Three elephants were seen fleeing to higher ground. On closer inspection, the elephants with tracking collars appeared to have exhibited normal movements for the day. If indeed animals sense danger from earthquakes or tsunamis, scientists do not know what it is they could be sensing, but they would like to find out.
Lesson Summary
[edit | edit source]- Seismologists use seismograms to determine how strong an earthquake is, how far away it is, and how long it lasts.
- Epicenters can be calculated using the difference in the arrival times of P-and S-waves from three seismograms.
- The intensity of an earthquake can be determined in many ways. The Mercalli Scale identifies the damage done and what people feel, the Richter Scale measures the greatest amplitude of the earthquake, and the moment magnitude scale measures the total energy released by an earthquake.
- Despite some successes, seismologists have not come too far in their ability to predict earthquakes.
Review Questions
[edit | edit source]- How can a seismograph measure ground shaking if all parts of it must be attached to the ground?
- On a seismogram, which waves arrive first, second, third and which arrive last?
- What information is needed for seismologists to calculate the distance that a seismic station is from an earthquake's epicenter?
- If a seismogram records P-waves and surface waves but not S-waves, where was the earthquake epicenter located relative to the seismograph and why?
- Like the Richter scale, the magnitude moment scale is logarithmic. What is the difference in the amount of energy released by an earthquake that is a 7.2 versus an 8.2 in magnitude? A 7.2 versus a 9.2?
- Why do you need at least three seismographs to locate an earthquake epicenter?
- While the Mercalli scale is still used for measuring earthquake magnitude, why is it not the only scale used? Where does it fall short relative to the Richter and Moment Magnitude scales?
- Why is the moment magnitude scale thought to be more useful than the Richter scale for measuring earthquake magnitudes?
- What is the difference in energy released between a 6 and a 7 on the Richter scale? How about a 6 and a 7 on the moment magnitude scale?
- How do seismologists use earthquake foreshocks to predict earthquakes? Why are foreshocks not always an effective prediction tool?
- What are the characteristics of a good earthquake prediction? Why are these features needed?
- Why were Chinese seismologists so successful at predicting the 1975 earthquake in the Manchurian province of Liaoning? Why did they fail to predict the 1976 Tangshan earthquake and evacuate Guandong Province a month later needlessly?
Vocabulary
[edit | edit source]- Mercalli intensity scale
- This scale measures the effects of an earthquakes seen on the land surface and felt by humans. It measures I-XII.
- moment magnitude scale
- This is a logarithmic scale that measures the total energy released by an earthquake. An increase of one integer indicates a 30-fold increase in energy released. An increase of two integers indicates a 1,000-fold increase in energy released.
- Richter scale
- The Richter scale measures the largest jolt produced by an earthquake. It is a logarithmic scale.
- seismogram
- A seismogram is the printed record of seismic activity produced by a seismometer.
- seismograph
- An older type of seismometer in which a pen that was suspended and weighted wrote on a drum that moved with the ground.
- seismometer
- A seismometer is a machine that measures seismic waves and other ground motions.
Points to Consider
[edit | edit source]- If you live in an earthquake prone area, how do you feel about your home now that you've read this section? Since earthquakes are unlikely to be predicted, what can you do to minimize the risk to you and your family? If you do not live in an earthquake prone area, what would it take to get you to move to one? Also, what risks from natural disasters do you face where you live?
- What do you think is the most promising set of clues that scientists might some day be able to use to predict earthquakes?
- What good does information about possible earthquake locations do for communities in those earthquake-prone regions?